CRISPR - What Is It, and How Can It Be Used In Medicine?
On December 8th, 2023, the FDA approved two new gene therapies for sickle cell disease. Exagamglogene Autotemcel (Casgevy), is the first CRISPR based theory to gain FDA approval. Using CRISPR/Cas9 technology the patient’s stem cells are modified and then reinfused back into the patient where they engraft (attach and multiply) in the patient’s bone marrow to increase the production of hemoglobin F.
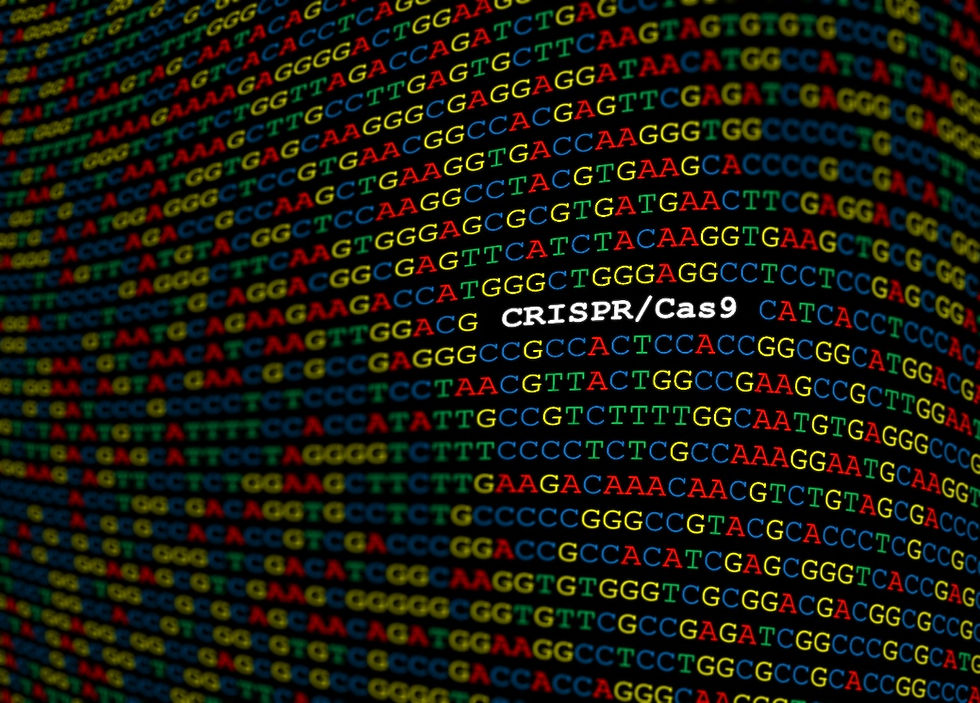
Updated December 19, 2023
The discovery of CRISPR, a gene editing technology, may turn out to be one of the most significant scientific advances in human history. We now have the ability to manipulate DNA relatively easily in ourselves and other animals, plants, and microscopic organisms. For the first time, humans can control their own genetic destiny. This article will delve into what CRISPR actually is, how it works, and what the ramifications are for disease treatment and society as a whole.
Jennifer Doudna, PhD and Emmanuelle Charpentier, PhD received the Nobel Prize in Chemistry in 2020 for their 2012 discovery of how to use CRISPR to edit the genome. Their work was truly groundbreaking and stood on the shoulders of other discoveries such as the structure of DNA by Watson and Crick, the Human Genomic Project which mapped out the complete human DNA, and previous researchers who discovered the CRISPR system and the nuclease enzyme Cas9 it uses to make cuts in genetic material.[1] Before CRISPR it was possible to make changes to DNA, but it was cumbersome, time consuming, and expensive. CRISPR made redesigning genetic material a much faster and easier process.
What is CRISPR in Nature?
CRISPR is an acronym for Clustered Regularly Interspaced Short Palindromic Repeats.[2] CRISPR is the process in nature by which a bacterial cell can identify, cut, and inactivate DNA sequences in viruses to protect itself from viral attacks. It was discovered by Francisco Mojica, PhD in 1993[1], who when sequencing bacterial DNA noticed that there were short segments of DNA that appeared to have no functionality, and were found at regular intervals. The segments were palindromic, meaning each side of the segment was a mirror image of the other side.
It was later discovered that these DNA segments were duplicates of short segments of the genetic code of DNA viruses (bacteriophages) that had previously attacked the bacteria. When a bacterium is attacked, it is able to cut off a segment of the viral DNA called a protospacer and then incorporate that section into its own DNA, at which time the new segment is called a spacer. The bacterium is then able to make inverse RNA copies of that DNA spacer which matches the viral DNA. Those inverse RNA copies can be transported and attached to the viral DNA by guide RNA(gRNA) where a nuclease enzyme called Cas9(CRISPR-associated protein 9) unzips the double stranded viral
DNA exposing the base pairs. After attachment, Cas9 acts like a DNA scissors cutting
both strands of the viral DNA, preventing the virus from replicating and protecting the
bacterium from further attack.[2,3,4]
The CRISPR process requires guide RNA(gRNA), a specific RNA sequence that identifies
the target DNA region of interest (such as in an invading virus). gRNA consists of two
components: 1) CRISPR RNA (crRNA), a 17-20 nucleotide sequence copied from the
bacterial DNA spacer that is complementary to the target viral DNA protospacer and 2)
transport RNA (tracrRNA), which carriers the Cas9 nuclease enzyme to the target viral
DNA.[4]
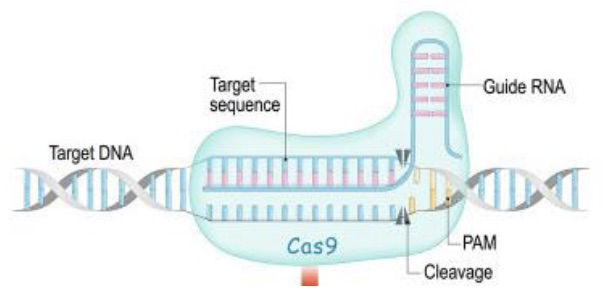
CRISPR Needs a PAM Sequence to Work
The Cas9 nuclease is carried by the gRNA to a specified portion of the target viral DNA. For CRISPR to work, there must be a Protospacer Adjacent Motif (PAM) sequence located near the targeted section of viral DNA. The PAM sequence is most typically two to six nucleotides in length, but can be longer. It serves as a signal or keyhole for Cas9 and is required for Cas9 activation that results in the cutting and inactivation of the viral DNA. The PAM sequence is generally found three to four nucleotides away from the cut site on the viral DNA.[3]
As the bacterial spacer contains the same gene base sequences as the virus, it is theoretically possible for the gRNA to mistakenly attach to the bacteria’s own DNA, and if activated result in its own destruction. The PAM sequence protects the bacteria from doing that, as the correct PAM sequence found on the virus is needed for the CRISPR-Cas9 system to activate.[3]
Once the Cas9 is attached to the correct viral DNA segment and has identified the proper PAM sequence, it makes a double-stranded break in the target DNA.[2,3,4] At that point, the DNA strand may reattach with some mutations that adversely affect it, or become nonfunctional, providing the bacteria protection against that viral DNA strand forming new viruses.[2]
CRISPR In the Laboratory
In the laboratory CRISPR can be used for gene editing. A specific gRNA is used and combined with a nuclease such as Cas9 with the needed PAM sequence. This is mixed with cells targeted for editing and after attachment of the gRNA, the Cas9 cleaves the target DNA. A new DNA gene or segment is can then be inserted into the cleaved DNA with reclosure of the cut DNA segments. CRISPR can be used to either induce or correct a mutation or remove a gene or set of genes from the targeted DNA. One technical limitation is that CRISPR, while much more accurate than other gene editors, can still at times cut in the wrong place, resulting in undesirable or unexpected effects.[2]
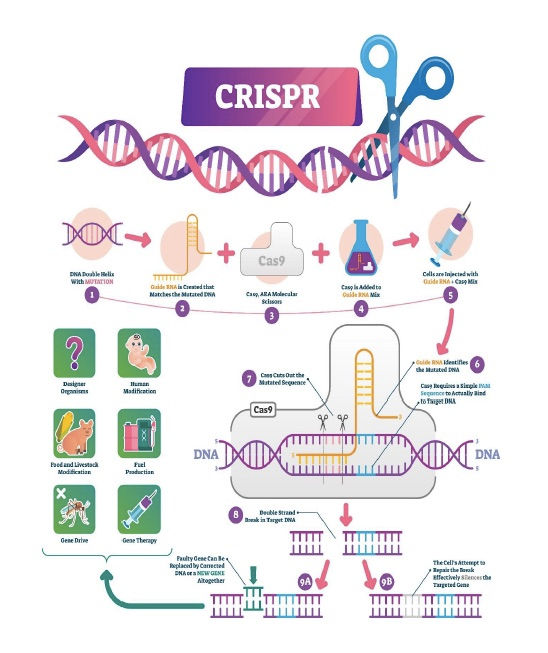
CRISPR Modifications
Single Guide RNA
Doudna and Charpentier were able to create a new type of RNA not found in nature, called single guide RNA (sgRNA), which is a single RNA molecule that contains both the crRNA and tracrRNA sequences. sgRNA being one molecule rather than the two separate ones found in nature made genetic manipulation in the laboratory much easier. Doudna and Charpentier received the Nobel prize for figuring out the specific mechanics of the CRISPR system, understanding that this method could be used to manipulate DNA in any living creature or plant, and creating sgRNA.[5]
CRISPR Improvements
The CRISPR concept, although groundbreaking, is now considered somewhat of a blunt instrument by scientists. CRISPR only works on DNA, and mutational errors are possible as both strands of the DNA try to repair themselves. In nature, once the viral DNA has been cleaved, the process is completed and the virus can no longer replicate. However, laboratory gene editing is different as when the Cas9-cleaved DNA tries to repair itself, the cell will try to repair the break using any available DNA it can find. Scientists can inject large amounts of the new gene they want to insert into the target cell in the hope that it will be inserted at the site of the DNA cut.[6]
The CRISPR system has been modified and improved for gene editing, so rather than just cutting the DNA it can also add activators or depressors of gene function to
specified areas, or edit a specific gene by changing one nucleotide for another.[2,6]
Scientists have created modified CRISPR-Cas9 to cut only one strand of DNA, not both,
to allow more pinpoint gene editing.[2] A newer technique is called prime editing, which is able to pinpoint and make small nucleotide base changes. It uses a modified Cas9 enzyme that initially just cuts one strand of DNA and replaces the targeted nucleotides with the new ones. Then the Cas9 nicks the complementary DNA strand and replaces the corresponding part, correcting both strands of DNA with much less chance of a mutation. This method could also allow changing genes in multiple locations for diseases such as Tay-Sachs.[7]
Scientists have also adapted CRISPR to edit RNA using a recently discovered different nuclease Cas13(CRISPR-associated protein 13) enzyme. This could expand CRISPR’s usefulness to modify RNA viruses, such as SARS-CoV-2 that causes COVID-19.[8]
Improved PAM Sequence Activation
In genetic editing the most commonly used Cas9 nuclease is derived from Streptococcus pyogenes.[3] PAM sequences for S. pyogenes that activate Cas9 are spread throughout the human genome (an estimated 161 million times or every 42 nucleotides[9]), but they are not always positioned in the correct location to activate gRNA to target a particular gene. If a PAM sequence is not located near a nucleotide
that is the target to be modified or removed, CRISPR may not work. Also, if the PAM sequence is located multiple times in the target DNA, there may be attachment of the gRNA to untargeted areas, and unwanted mutations may occur.
Genetic targeting in the laboratory can be improved by the use of a differently coded PAM sequence that may solely be located in the area of the targeted gene. This may be achieved by the use of S. pyogenes Cas9 variants that have different PAM sequences, using Cas9 nucleases derived from other bacterial species, or the use of more recently discovered non-Cas9 enzymes that have different PAM sequences and can also be used for genetic manipulation.[10]
The specific PAM sequence that activates Cas9 to cut the viral DNA for S. pyogenes is NGG where N is any nucleotide.* Just by chance this sequence is randomly found frequently in human DNA. S. pyogenes Cas9 variants have different PAM sequences such as NGAN, NGNG, NGAG, or NGCG which can be used to target different areas on the DNA chain. The PAM sequence for Staphylococcus aureus Cas9 is NGRRT, Neisseria meningitidis Cas9 has a NNNNGATT PAM sequence code. One can appreciate how by using different nucleases with different PAM codes, a Cas9 or non-Cas9 enzyme can be selected that will only activate near the specific target area you wish to modify.[10]
*(G= guanine, A=adenine, C=cytosine, N=any nucleotide, R= any purine(A or G)
CRISPR Ethical Considerations
Given that CRISPR has the potential to change a species forever, international meetings of geneticists were held on the ethics of how to use it for human gene editing. It was agreed that somatic cell manipulation was allowed in individual humans to help cure a disease, but more research was needed before germ cell manipulation was to be attempted which would be passed on to progeny.[5]
However, in 2018 a Chinese geneticist He Jiankui, PhD modified embryos which were then implanted and led to the birth of twin girls. He disabled a gene called CCR5, which encodes a protein that allows HIV to enter cells, in an attempt to mimic a mutation that exists in about 10% of Europeans and protects them from HIV infection. However, as CRISPR gene editing had never been done before in human germ cells that were used for conception, there was no way of knowing if he also induced other mutations in the
girls’ genomes that might affect them adversely. Ironically, CCR5 is thought to actually help people fight off the effects of various other infections, such as West Nile virus and the girls might then be more susceptible to other diseases as well.[11] In December 2019, Dr. Jiankui was sentenced to three years' imprisonment and a three-million-yuan fine(approximately $450,000) for this banned human research.[12]
Medical Uses of CRISPR
Sickle Cell Disease and Thalassemia
The initial uses of CRISPR to fight disease involved attempts to cure patients with genetic diseases through simple gene substitutions. Sickle cell anemia and beta thalassemia are some examples. It has been found that inducing sickle cell and thalassemia patients to produce higher levels of fetal hemoglobin (hemoglobin F) instead of hemoglobin S will reduce their symptoms. After birth, fetal hemoglobin
production is normally reduced by the BCL11A locus on chromosome 2, which is the target gene scientists were attempting to manipulate using CRISPR technology.[13] In one study of two patients, one with sickle cell anemia and one with beta-thalassemia, CD34+ hematopoietic stem and progenitor cells were harvested from the patients by apheresis. (Separation of withdrawn blood into blood components followed by the retransfusion of only some of the blood components back into the patient.) The cells were then edited by CRISPR-Cas9 technology to reduce the BCL11A inhibition which would have the desirable effect of increasing hemoglobin F production. Myeloablative conditioning was performed on the patients to allow these new cells to graft into the bone marrow, and then the cells were reinfused. The patient with sickle cell disease had averaged seven severe vaso-occlusive episodes per year prior to therapy, and at 16
months after therapy had not had another one. The thalassemia patient, who had averaged 34 units of packed red cells per year prior to therapy, received only one transfusion of packed red cells 30 days after the infusion and after that did not require any more as of 18 months of follow-up. Both patients had serious infections soon after the myeloablative conditioning/infusions which were treated successfully.[14]
Another method recently demonstrated experimentally in mice is using a direct gene edit. Sickle cell disease is the result of a one base pair mutation in the gene that controls hemoglobin production. The sickle hemoglobin allele was converted to a normal allele in stem cells taken from mice using CRISPR and then transfused back into the mice after they had been irradiated to destroy their existing bone marrow cells. It was found that after exposure to hypoxic conditions only 29.8% of the RBCs in the genetically treated mice sickled, compared to 86.3% in the control group. Spleen size was also noted to be smaller in the treated mice.[15]
It should be noted that there are other non-CRISPR methods being tested and used to try to cure thalassemia and sickle cell disease. One promising method, lentiglobin gene therapy uses a virus vector to seed the bone marrow with RNA that increases expression of the hemoglobin F gene.[16] However, some scientists are wary of using lentiviruses for gene therapy as integration into the genome is random, and because of their potential to cause unintended disruptions in other genes.[13]
Update (December 19, 2023)
On December 8th, 2023, the FDA approved two new gene therapies for sickle cell disease. Exagamglogene Autotemcel (Casgevy), is the first CRISPR based theory to gain FDA approval. Using CRISPR/Cas9 technology the patient’s stem cells are modified and then reinfused back into the patient where they engraft (attach and multiply) in the patient’s bone marrow to increase the production of hemoglobin F. In a study of 44 patients, in which 31 made inclusion criteria, it was reported that 93.5% had been free of severe vaso-occlusive crises for at least one 12 consecutive month period over 2 years.[U1]
The second approval was for lovotibeglogene autotemcel (Lyfgenia), a cell-based gene therapy. Lovotibeglogene autotemcel does not use CRISPR technology, but uses a lentiviral vector to seed the bone marrow. With lovotibeglogene autotemcel, the patient’s stem cells are genetically modified to produce HbA-T87Q, a gene-therapy derived hemoglobin which functions similarly to hemoglobin A.[U1] In a study of 47 patients, in which 34 made inclusion criteria, 88% were vaso-occlusion event free and 94% were severe vaso-occlusion event free for a median of 35.8 months.[U2]
In addition to the typical hematological adverse events associated with myeloablation required for stem cell therapy, several patients in clinical trials getting lovotibeglogene autotemcel, developed a hematological malignancy. There is a black box warning that recommends life-long monitoring of patients who receive lovotibeglogene autotemcel for malignancy.[U3]
The number of patients getting these new therapies may be limited by the ability of patients to withstand myeloablation that is needed before the therapy can be given, the need for a month-long hospitalization, a limited number of medical centers approved to administer the therapies and a cost of 2.2 to 3.1 million dollars per patient. In addition, the long-term effects of these therapies are not known yet.[U4]
As of August 17, 2022, there is also an FDA approved cell-based gene therapy for beta thalassemia called betibeglogene autotemcel (Zynteglo). It is similar to lovotibeglogene autotemcel and engrafts in the patient’s bone marrow to cause production of beta A-T87Q-globin (a modified beta-globin protein) to replace the patient’s damaged beta-globin. The new beta-globin combines with alpha-globin to produce functional adult hemoglobin. There is no black box cancer warning for betibeglogene autotemcel, but cancer screening for at least 15 years is recommended.[U5,U6]
Leber’s Congenital Amaurosis 10
Another recent use of CRISPR is treating Leber’s congenital amaurosis 10 (LCA 10). No treatment is currently available for the disease, which is a cause of blindness in childhood. Mutations in the CEP290 gene disable photoreceptors in the retina, although the cells are still present and potentially functional in people with LCA10. CRISPR edited genes were experimentally injected into one eye near the retina of some subjects in hopes that the mutations in CEP290 could be replaced locally with a normal CEP290 gene and the photoreceptors turned back on.[17] Although results are not published yet, two of the patients said in an interview that their vision has not been fully restored, but they are able to see colors and make out shapes of objects much better than before the therapy. For one patient the therapy improved his ability to feed himself as he was able to see both eating utensils and his food better. However, for as
yet unclear reasons, the therapy has not worked for all the patients in the study.[18]
Other Potential Medical Uses
CRISPR is also being studied for targeted cancer therapy[19], but in the future, people or embryos with genetic mutations that increase cancer risk might be able to have those genes modified. There are probably many other diseases that could eventually be treated using CRISPR technology, such as targeting an infecting organism’s ability to replicate or the body’s response to it.
Non-Medical Uses of CRISPR for Disease Control[20,21]
Gene drives are genetic modifications in the germ cells designed to spread through a population at higher-than-normal rates of inheritance. Since 2014, scientists have engineered CRISPR-based gene drive systems in mosquitoes, fruit flies, and fungi, and are currently developing them in mice.
Scientists use CRISPR to insert the selected gene drive in both strands of DNA in an animal. After that, all the offspring of a mating between a modified and unmodified animal will have the gene drive on one chromosome while the other chromosome will be normal. During early development of the offspring, the CRISPR portion of the gene drive activates and cuts the normal gene. The gene drive then inserts a copy of itself into the normal chromosome making the progeny homozygous for the gene drive and ensuring nearly 100% transmittal of the gene drive to the next generation.
One problem with gene drives is that genetic variation may confer resistance to the gene drive, which has occurred frequently in fruit fly experiments. Mutations may also occur that alter the genetic sequence CRISPR is set to recognize, preventing the gene from being edited. To counteract this problem, scientists are focusing on certain genes that seem to be more protected against mutation. One such gene in mosquitoes is called doublesex, and controls differentiation of the sexes. With a gene drive targeting the doublesex gene, modified female mosquitoes were unable to bite or lay eggs. In the laboratory it was found that in 8 to 12 generations the mosquito population was effectively terminated.
There is currently interest in gene drive research to have mosquitoes produce an antibody that inactivates an infectious agent, such as the dengue virus, so the mosquito cannot pass the disease on to humans. Another gene drive is being investigated that causes an internal toxin to be released that will kill a mosquito if it gets infected with a pathogen dangerous to humans.
An issue with using gene drive genetic manipulation is that you can potentially change a species forever. One way of preventing that is to develop gene drives that are self-extinguishing. Scientists can engineer a gene drive to gradually lose the ability to propagate, until it runs out over several generations and is unable to spread beyond a target population of mosquitoes or flies. Another method is to use a gene drive that
requires continual release of genetically modified mosquitoes or flies for many generations. When those releases stop, the modification becomes diluted with wild-type versions of the gene and is extinguished within a few years.
Unfortunately, the future effects of genetic modification are unknown. In trying to eliminate a disease the organism may mutate and become more virulent or find another host. Eliminating or modifying a species may also have unintentional negative effects on the ecology of an area.
Ecological Uses of CRISPR
Non-medical uses of CRISPR are being tested in many ecological areas. CRISPR is being tested to improve outputs of yeast, bacteria, or algae created biofuels.[22,23] Attempts are being made to modify bacteria and fungi that can degrade plastic, in an attempt to eliminate the significant ecological problem of plastic waste. Current projects are underway using CRISPR to engineer plants or bacteria to improve nitrogen fixation to reduce nitrogen runoff from the soil in farms, which can contaminate water sources. It
also can be used to modify crops or animals to intensify or diminish certain traits, such as modifying crops to be resistant to insects, which could potentially reduce pesticide use.[23]
The Future
The future in this new era of genetic manipulation is uncertain. CRISPR derived technology is very powerful and may do enormous good, potentially ending the suffering that comes with some genetic diseases, cancer, and infectious diseases. Just ending mosquito borne malaria could potentially prevent over 400,000 deaths and close to 229 million infections each year.[24]
On the other hand, CRISPR technology may lead to increasing wealth and societal disparity as some sectors of society may be able to genetically enhance their offspring, while those with less access to the technology may not have that opportunity. Problematic issues include the possibility of a well-intentioned genetic manipulation in an insect leading to ecological disaster, or rogue scientists or nations creating entirely new animal species or novel types of humans.
At the start of this age of advanced genetic manipulation, we are all living in a huge experimental laboratory. Only time will tell how it works out.
“It is not in the stars to hold our destiny but in ourselves.” -William Shakespeare
References
[1] CRISPR Timeline. Broad Institute. 2021. Retrieved from: www.broadinstitute.org/what-broad/areas-focus/project-spotlight/crispr-timeline
[2] CRISPR Explained. Novateinbio. 2019. www.novateinbio.com/content/96-crispr-explained
[3] Importance of the PAM Sequence in CRISPR Experiments. Synthego. 2021. Retrieved from: www.synthego.com/guide/how-to-use-crispr/pam-sequence
[4] The Complete Guide to Understanding CRISPR sgRNA. Synthego. 2021 Retrieved from: www.synthego.com/guide/how-to-use-crispr/sgrna
[5] Isaacson W, The Codebreaker. Simon & Schuster. March 9, 2021.
[6] Crossley M. What is CRISPR gene editing, and how does it work? The conversation. January 31, 2018. Retrieved from: www.theconversation.com/what-is-crispr-gene-editing-and-how-does-it-work-84591
[7] Super-precise new CRISPR tool could tackle a plethora of genetic diseases. Nature. 21 October 2019. Retrieved from: www.nature.com/articles/d41586-019-03164-5
[8] New kind of CRISPR technology to target RNA, including RNA viruses like coronavirus. Science Daily. March 16, 2020. Retrieved from: www.sciencedaily.com/releases/2020/03/200316141514.htm
[9] Integrated DNA Technologies. 2021. Retrieved from: www.idtdna.com/pages/support/faqs/how-often-are-the-pam-sequences-presented-in-the-mammalian-genome-in-average
[10] McDade J. The PAM Requirement and Expanding CRISPR Beyond SpCas9. Addgene. Updated Aug 20, 2020. Retrieved from: www.blog.addgene.org/the-pam-requirement-and-expanding-crispr-beyond-spcas9
[11] Cyranoski D. The CRISPR-baby scandal: what’s next for human gene-editing. Nature. 26 February 2019. Retrieved from: www.nature.com/articles/d41586-019-00673-1
[12] Wee SL. Chinese Scientist Who Genetically Edited Babies Gets 3 Years in Prison. The New York Times. Dec. 30, 2019. Retrieved from: www.nytimes.com/2019/12/30/business/china-scientist-genetic-baby-prison.html
[13] Michael Eisenstein. Gene therapies close in on a cure for sickle-cell disease. Nature. 25 August 2021. Retrieved from: www.nature.com/articles/d41586-021-02138-w
[14] Frangoul H et al. CRISPR-Cas9 Gene Editing for Sickle Cell Disease and β-Thalassemia. N Engl J Med 2021; 384:252-260. January 21, 2021. Retrieved from: www.nejm.org/doi/full/10.1056/NEJMoa2031054
[15] Newby, G.A., Yen, J.S., Woodard, K.J. et al. Base editing of haematopoietic stem cells rescues sickle cell disease in mice. Nature 595, 295–302 (2021). Retrieved from: www.nature.com/articles/s41586-021-03609-w'
[16] Thompson AA et al. Lentiglobin Gene Therapy for Transfusion-Dependent β-Thalassemia: Update from the Northstar Hgb-204 Phase 1/2 Clinical Study. Blood, volume 128, Issue 22. December 2, 2016. Retrieved from: www.ashpublications.org/blood/article/128/22/1175/96014/Lentiglobin-Gene-Therapy-for-Transfusion-Dependent
[17] Ledford H. CRISPR treatment inserted directly into the body for first time. Nature. 05 March 2020. Retrieved from: www.nature.com/articles/d41586-020-00655-8
[18] Stein R. A Gene-Editing Experiment Let These Patients With Vision Loss See Color Again. NPR-WNYC. September 29, 2021. Retrieved from: www.npr.org/sections/health-shots/2021/09/29/1040879179/vision-loss-crispr-treatment?t=1633005081997
[19] How CRISPR Is Changing Cancer Research and Treatment. National Cancer Institute. July 27, 2020. Retrieved from: www.cancer.gov/news-events/cancer-currents-blog/2020/crispr-cancer-research-treatment
[20] Scudellari M. Self-destructing mosquitoes and sterilized rodents: the promise of gene drives. Nature. 09 July 2019. Retrieved from: www.nature.com/articles/d41586-019-02087-5
[21] Kyrou, K. et al. A CRISPR–Cas9 gene drive targeting doublesex causes complete population suppression in caged Anopheles gambiae mosquitoes. Nat Biotechnol 36, 1062–1066 (2018). Retrieved from: www.nature.com/articles/nbt.4245.pdf
[22] Javed MR et al. Current situation of biofuel production and its enhancement by CRISPR/Cas9-mediated genome engineering of microbial cells. Microbiological Research, Volume 219, Pages 1-1. 2019. Retrieved from: www.sciencedirect.com/science/article/pii/S0944501318308346?via%3Dihub
[23] Gallegos J, 10 ways CRISPR will revolutionize environmental science. Alliance for Science. July 17, 2018. Retrieved from: www.allianceforscience.cornell.edu/blog/2018/07/10-ways-crispr-will-revolutionize-environmental-science/
[24] World Malaria Report 2020-20 years of global progress & challenges. World Health Organization. 30 November 2020. Retrieved from: www.who.int/publications/i/item/9789240015791
[U1] FDA Approves First Gene Therapies to Treat Patients with Sickle Cell Disease. FDA website. December 8th, 2023. Retrieved from: https://www.fda.gov/news-events/press-announcements/fda-approves-first-gene-therapies-treat-patients-sickle-cell-disease
[U2] Long-term Follow-up Data From bluebird’s Gene Therapy Program in Sickle Cell Disease Support Durable, Potentially Curative Benefits Through Stable Production of Anti-Sickling Adult Hemoglobin and Resolution of Vaso-Occlusive Events. Bluebird Bio. Retrieved from: https://investor.bluebirdbio.com/news-releases/news-release-details/long-term-follow-data-bluebirds-gene-therapy-program-sickle-cell
[U3] LYFGENIA (lovotibeglogene autotemcel) prescribing information. FDA website. Revised 12/23. Retrieved from: https://www.fda.gov/media/174610/download
[U4] Kolata g. F.D.A. Approves Sickle Cell Treatments, Including One That Uses CRISPR. The New York Times. December 8, 2023. Retrieved from: https://www.nytimes.com/2023/12/08/health/fda-sickle-cell-crispr.html
[U5] ZYNTEGLO (betibeglogene autotemcel) prescribing information. Bluebird Bio.com. August 2022. Retrieved from: https://www.bluebirdbio.com/-/media/bluebirdbio/Corporate%20COM/Files/Zynteglo/ZYNTEGLO_prescribing_information.pdf
[U6] FDA Approves First Cell-Based Gene Therapy to Treat Adult and Pediatric Patients with Beta-thalassemia Who Require Regular Blood Transfusions. FDA website. August 17, 2022. Retrieved from: https://www.fda.gov/news-events/press-announcements/fda-approves-first-cell-based-gene-therapy-treat-adult-and-pediatric-patients-beta-thalassemia-who
initially published: 11/19/2021
Comments